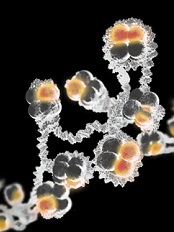
Credit: Eric Smith
Investigators have uncovered an unanticipated mechanism underlying trimethylation of a histone that activates gene expression.
And this finding could have implications for the treatment of leukemias and lymphomas.
Ali Shilatifard, PhD, of the Stowers Institute for Medical Research in Kansas City, Missouri, and his colleagues described the discovery in Genes & Development.
Histones, which come in 4 subtypes—H2A, H2B, H3, and H4—can either coil DNA into inaccessible, silent regions or untwist it to allow gene expression. And small chemical flags, such as methyl groups, affect whether histones silence or activate genes.
Among activator histones is a form of H3 decorated at a precise location with 3 methyl groups, known as H3K4me3.
Previous research showed that the presence of H2B exhibiting a single ubiquitin molecule stimulated the methylase that modifies H3K4, thereby increasing H3K4me3 levels.
But how the methylase’s activity was directed toward the appropriate targets was unclear.
Now, Dr Shilatifard and his colleagues have discovered a mechanism underlying H3K4 trimethylation. Their research explains why H3K4me3 is deposited adjacent to a target gene promoter rather than haphazardly across the entire gene.
The team said this finding is significant because mutations in the human gene encoding the methylase responsible for H3K4me3 are associated with leukemias, lymphomas, and other malignancies.
The methylase in question, named SET1 in yeast and MLL in mammals, is part of a protein aggregate called COMPASS (COMplex of Proteins ASsociated with Set1). Dr Shilatifard was the first to define the role of COMPASS in chromatin modification.
“Over a decade ago, our lab used yeast to show that COMPASS was an H3 methylase,” he said. “Since these fundamental systems are highly conserved from yeast to Drosophila to humans, we took advantage of the awesome power of yeast genetics to identify what regulates H3K4 methylation activity.”
Part of his group’s latest paper addresses SET1/MLL regulation by different proteins within yeast COMPASS.
The investigators knew that if more than half of SET1’s front end was removed, levels of DNA-bound trimethylated H3K4 in cells harboring the remaining “stub” were equal to those in cells containing the full-length protein when analyzed in bulk.
This finding led some researchers to presume that the entire front end of SET1/MLL, as well as factors that interact with it, must not be needed to regulate H3K4me3 activity.
But Dr Shilatifard and his colleagues found evidence suggesting this presumption is incorrect.
The team first employed biochemical methods to capture every piece of DNA bound to H3K4me3 in the genome of yeast harboring either full-length SET1 or the stub missing the front end. They then sequenced all of those DNA fragments and mapped their position in the yeast genome.
Results showed that even though H3K4me3 levels in bulk were equivalent in normal and mutant cells, H3K4me3 was differentially distributed throughout the genome.
In normal cells, H3K4me3 complexes sat primarily on DNA promoter regions. By contrast, the DNA of cells harboring the stub exhibited DNA-binding H3K4me3 complexes in the middle of or between genes.
The work shows that COMPASS factors that bind to the SET1/MLL front end limit H3K4me3 deposition to the correct genomic sites (the promoter regions), while factors that bind the SET1/MLL stub increase the protein’s half-life.
The investigators also discovered how H2B ubiquitin modification machineries stimulate the entire process.
The team said understanding COMPASS regulation is essential, as genes encoding factors in the complex are mutant in numerous cancers.
